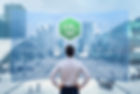
The fight against global warming is waging on many fronts. The drive to end our dependence on fossil fuels by converting to renewable power, electric vehicles, and energy efficiency continues to gain momentum and capture the attention of a concerned global populace.
In our ongoing efforts, all options are on the table. Even in an ideal scenario in which everyone is driving EVs, all buildings are efficient, and most rooftops are equipped with solar panels, industrial and manufacturing activities will continue to produce CO2 and other greenhouse gases. On top of these emissions, the many megatons of gases emitted by more than a century of commercial and industrial activity will remain trapped in our atmosphere and continue their warming effect.
What other steps can the world take to minimize or offset these emissions and remove existing gases from the atmosphere?
Fortunately, a myriad of technologies have been developed. Just as we have figured out how to power our homes and vehicles without harmful emissions, we have also devised ways to perform the less visible but equally valuable process of capturing carbon from their sources and from the atmosphere, then putting it back into the ground or into other stable uses, where it can do no harm.
Carbon Capture, Utilization and Storage (CCUS)
Various methods are in use to capture carbon dioxide gas and store it someplace other than in the atmosphere. Collectively, they are referred to as Carbon Capture, Utilization and Storage (CCUS). According to the International Energy Agency (IEA), a global organization that conducts research and fosters dialogue among nations about energy policy, CCUS refers to a "suite of technologies." They capture carbon from industrial processes or power generation, or directly from the atmosphere, then transport it for use or storage.
CCUS can be broken down into a few categories. One of the more recognized methods is point source carbon capture. The Massachusetts Institute of Technology defines point source carbon capture as methods of collecting carbon dioxide (CO2) directly from power plants to lower their emissions. A power plant may be entirely rebuilt with the new technology, or it may be "retrofitted" by adding the technology to an existing facility.
Not all direct source carbon capture involves power plants. Many industrial processes, such as the production of chemicals, iron, steel, and cement, also produce CO2. The U.S. Department of Energy's National Energy Technology Laboratory notes that the U.S. industrial sector accounted for over 1,300 million metric tons of CO2 emissions in 2020.
Targeting the cement industry will be a key strategy for the reduction of industrial emissions. Cement, one of the world's most widely consumed products, is a major source of CO2.
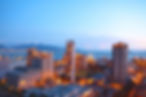
The journal Nature notes that 30 billion tons of concrete is used each year on a global scale. More importantly, at least 8% of global emissions caused by humans comes from the cement industry.
In cement manufacturing, CO2 is primarily released during the phase known as cement clinker production, which is done through a limestone calcination process. Methods are in use to capture these emissions. Direct separation involves the capture of CO2 emissions by indirectly heating the limestone. The technology strips CO2 directly from the limestone, without mixing it with other combustion gases, making it easier to capture.
Putting Technology into Action
Direct source technology has been deployed around the world to capture carbon emissions from the generation of power and from the manufacturing process for cement and other products. The Center for Climate and Energy Solutions (C2ES) notes that as of 2020, no fewer than 26 commercial-scale carbon capture projects were operating around the world with thirty-four more in various stages of development.
In 2014, for example, the SaskPower Boundary Dam project in Saskatchewan, Canada, became the first commercial-scale retrofit of an existing coal-fired power plant with carbon capture technology. The captured carbon is sold locally to extraction companies to be injected into the ground and used for oil extraction in a process known as Enhanced Oil Recovery (EOR). SaskPower boasts that the project is capable of reducing the CO2 emissions from the coal process by up to 90%, and that the facility captured 4,167 tons of carbon dioxide in January of this year, the most recent month for which figures are available.
Growing Plants to Capture Carbon
When it comes to some of society's most vexing problems, nature often reminds us that sustainable solutions are in plentiful supply and easy to harness. Take solar and wind power, for example.
The challenge of capturing carbon is no exception. One of the first things we learn in basic biology is that humans breathe air and exhale CO2, while plants do the opposite, exhale air and breathe in CO2.
This unique law of the natural world has not been lost on those pursuing technology to capture carbon. The simplest solution, of course, is to plant more plants. Reforestation, which is the planting of trees on land where they have been removed, and afforestation, which is the planting of trees on land where they have not historically existed, are legitimate approaches to increasing greenery on the planet to absorb more carbon from the atmosphere.
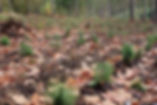
Bioenergy with carbon capture and storage (BECCS) takes the process one step further, by employing the carbon-absorbing properties of plants in the production of energy. It represents another effective capture method within the suite of CCUS technologies.
According to the American University in Washington D.C., BECCS can be broken down into two steps or phases. In the first phase, organic material or biomass (plants) is converted into heat, electricity, or fuels. In other words, it is consumed to create energy. In the second phase, the carbon emissions from this bioenergy conversion are captured and stored in geological formations or embedded in long-lasting products.
Bioenergy with carbon capture and storage employs the carbon-absorbing properties of plants in the production of energy.
This process takes advantage of the unique role of plants as natural consumers of carbon from the atmosphere as they grow, giving BECCS the ability to be a negative emissions technology, meaning it can remove more carbon that it emits. In this way, BECCS could serve to draw down the concentration of CO2 in the atmosphere. A recent study from the U.S. National Academy of Sciences estimates a global potential to sequester 3.4–5.2 gigatons (Gt) of CO2 per year via BECCS.
The technology is catching on and has been implemented in several instances. Perhaps the best example may be the Illinois Industrial Carbon Capture & Storage Project in Decatur, owned by the Archer Daniels Midland (ADM) Company. The project captures carbon dioxide from an ethanol production facility and sequesters it in a nearby deep saline formation. The project can capture up to 1.1 million tons of carbon dioxide per year.
Taking Carbon Out of the Air
Yet another capture technology may seem somewhat far-fetched. Capturing carbon directly out of the atmosphere sounds like the stuff of science fiction or the product of a vivid imagination. Make no mistake, it is real.
The IEA defines direct air capture (DAC) as technologies that extract CO2 directly from the atmosphere. The process works in one of two ways. One method involves liquid systems, which pass air through chemical solutions (e.g., a hydroxide solution), that removes the CO2. The system reintegrates the chemicals back into the process by applying high-temperature heat while returning the rest of the air to the environment.
Solid DAC technology makes use of solid sorbent filters that chemically bind with CO2. When the filters are heated and placed under a vacuum, they release the concentrated CO2, which is then captured for storage or use.
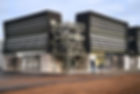
The IEA reports that there are currently nineteen direct air capture (DAC) plants operating worldwide. Collectively, they are capturing more than 0.01 megatons (Mt) of CO2 per year. The latest plant to come online, Climeworks' Orca plant in Hellisheidi, Iceland, near Reykavík, is the world's largest. The facility consists of eight collector containers, with an annual capture capacity of 500 tons each. It came online in September 2021 and is capturing about 4 kt of CO2 per year.
Where Does it All Go?
All this brilliant technology raises another very important question: Where does all the carbon go once it is captured?
Storage and utilization technologies address this problem. Captured carbon can be stored and used in several useful ways.
The C2ES notes that carbon dioxide can be injected into geological formations and stored deep underground. These formations offer vast untapped potential for storage of carbon emissions from centuries of fossil fuel use.
Options include the previously mentioned EOR, which uses injecting carbon dioxide to facilitate the extraction of oil and gas from existing sites. Oil and gas reservoirs have demonstrated their ability to store CO2 because they have held oil and gas resources in those same locations for millions of years.
Other types of geological features such as deep saline formations, coal beds, basalt formations, and shale basins also have the potential to store captured CO2 deep underground.
Not all carbon has to be stored underground. It can also be put into other materials that we encounter in our above-ground activities. For example, carbon can be stored in cement. Carbon mineralization injects captured CO2 into fresh concrete where it becomes permanently embedded and actually helps improve its strength.
In Redding, California, two companies teamed up to construct and operate a small commercial cement plant that will capture CO2 from the kiln exhaust and convert it into a cementitious material that will be used to produce high-quality concrete. The facility is a collaboration of the construction materials supplier, Lehigh Hanson, Inc., and the Silicon Valley-based materials technology company, Fortera.
CO2 can also be used to create other fuels that do not have their own carbon emissions. A facility in Sweden, owned by LiquidWind, will capture waste carbon dioxide and combine this with hydrogen, made from renewable electricity and water, to produce renewable methanol, e-methanol.
The Touchstone Research Laboratory in Triadelphia, West Virginia, will feature enclosed raceway ponds where algae will be cultivated using captured CO2. The algal lipids will be recovered to produce biofuel and the algae biomass will be used in an anaerobic digestion process to produce electricity and recover nutrients.
Challenges and Opportunities
The possibilities for capturing, storing, and using carbon dioxide are plentiful, but challenges abound. The most formidable challenge is the costs. According to the IEA, carbon capture can cost anywhere from $15 to $120 per ton of captured carbon.
Opponents also argue that capturing and sequestering carbon, or using it to extract more fossil fuels, is not the right strategy to combat global warming. Only the complete elimination of activities that burn fossil fuels will allow the planet to meet its targets for reducing greenhouse gas emissions and stabilizing global temperatures.
On the other hand, the fight against global warming is an all-encompassing challenge. All tools should be utilized. For many industries and specific facilities, other options may not be feasible. Capturing and selling carbon as a useful product creates a revenue stream that makes the technology more feasible. In those instances where reusing carbon is not feasible or desirable, regulatory penalties for carbon emissions could provide the necessary incentive.
In either case, CCUS allows for a flexible transition and conversion to a greener planet. It should be considered and cultivated along with other options.
*Rick Laezman is a freelance writer in Los Angeles, California, US. He has a passion for energy efficiency and innovation. He has been covering renewable power and other related subjects for more than ten years.